Waves
Gary Griggs | Distinguished Professor of Earth Sciences | University of California Santa Cruz
Introduction
Waves and beaches are inextricably connected, tied at the hip, so to speak. Without waves we would have no beaches. They are the single most important process affecting beaches and coastlines around the planet. There is no question that the underlying geology and tectonic setting of any coastal region has produced the large-scale landforms, such as the mountains, the cliffs, coastal plains, and uplifted marine terraces, but that’s long-term. It is the waves that impact and shape and reshape the coastline and beaches day in and day out, winter and spring, summer and fall.
There are a many different kinds of waves out there in the ocean, some we can see, and others we cannot. The tides that slosh back and forth from one side of the ocean to the other, once or twice a day in response to the gravitational pull of the moon and the sun are one kind of very long wave. Large disruptions or displacements of the seafloor, by undersea earthquakes, volcanic eruptions, or landslides, generate another type of very fast-moving wave, which we recognize as tsunamis or seismic sea waves, that can be disastrous for coastal cities and communities.
Wind Waves: Their Formation, Dispersal and Importance to Shorelines and Beaches
The waves we see breaking on the shoreline, those most visible to us and the ones that make surfing possible, are generated by the wind. No matter where we are along the coast, there are waves breaking on the beach arriving from somewhere; they might have been generated by the afternoon wind a few miles offshore or may have traveled thousands of miles from a distant storm many days earlier. All we need to begin to make waves in the ocean is some wind. Just like you can create small ripples or waves in your cup of coffee or tea as you blow to cool it down, the wind accomplishes the same thing on a much larger scale on the ocean surface.
When the wind first begins to blow across the sea surface, the friction of the air moving across the water will create very small ripples, sometimes called cat’s paws. Once a ripple has formed, there is now a steeper side against which the wind can exert more pressure. This allows the energy of the wind to be more effectively transmitted to the water surface and the ripples can begin to grow into small waves. As long as the wind continues to blow, more energy can be transferred to the sea surface and the waves can increase in size. Because the wind doesn’t blow at a completely uniform velocity and because the sea surface is rarely smooth, the pattern of waves initially created will be somewhat irregular and choppy. If we were at sea in a ship during a storm, we would see small and large waves, some forming as we watched and others that were passing by the ship, having been formed miles away by some other storm. This irregular mixture of waves of different heights and lengths is referred to by oceanographers as sea.
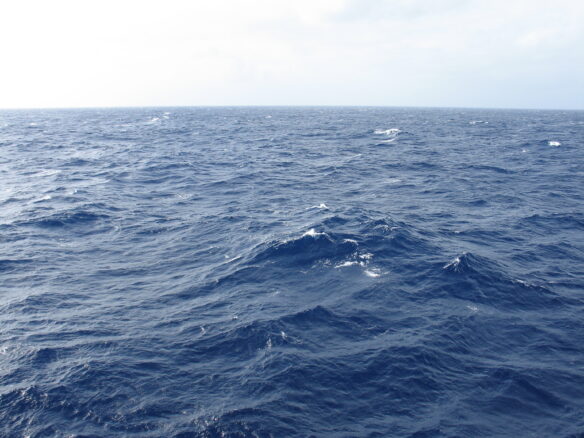
If the wind continues blowing in the same general direction long enough, the waves will begin to travel in that direction and sort themselves out. As the waves increase in size, having been given more energy by the wind, we can begin to recognize them as individual features with some recognizable properties. Each wave has a crest and a trough, and can be defined with a height, a wavelength, a period and a velocity, or speed. When the waves leaving a storm area have sorted themselves out and are more uniformly shaped, we refer to this condition as swell. You will often hear on the weather channel, or when coastal wave conditions are being described, that there is a “six-foot swell at 11 seconds and a local two-foot sea.” The swell has come from much farther away and is more uniform while the sea is generated by the local winds and is usually more irregular and much smaller.
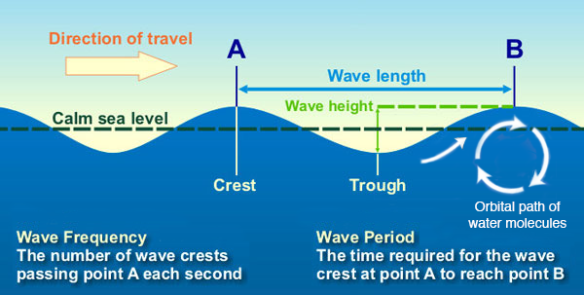
Wave period is expressed in seconds and is simply the time it takes for two successive wave crests or troughs to pass a single point. If you are standing on a pier, for example, you can look down and watch the waves passing beneath you. If you looked at your watch and counted now many seconds it took for two wave crests to pass the same piling, you would have measured the wave period. Most of the waves we see breaking along our coasts are commonly in the five to ten or twelve second range. There are many locally generated waves that have shorter periods, and we will also see waves approaching the shoreline that have traveled thousands of miles that have periods of 15 seconds or longer.
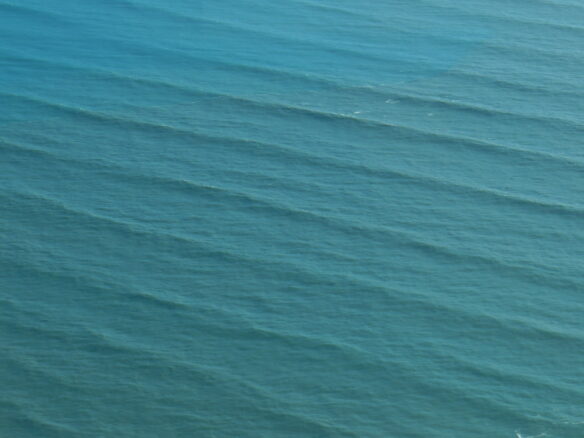
Wavelength is just the distance between two successive crests and is usually listed in feet or meters. If you throw a stone into a pond or lake you will create a set of waves that move out in a circular pattern from where the stone hit the water and they will have wavelengths we can measure in inches. Waves far out at sea or even those that approach the shoreline will usually have wavelengths of hundreds or even thousands of feet.
The height of a wave is just the vertical distance, usually given in feet, from the crest or highest point on the wave to the trough or lowest point. Ripples or very small waves a few inches in height form in a lake or pond, but surfers don’t usually begin to get excited or interested until the waves get up to 5 or 6 feet or more.
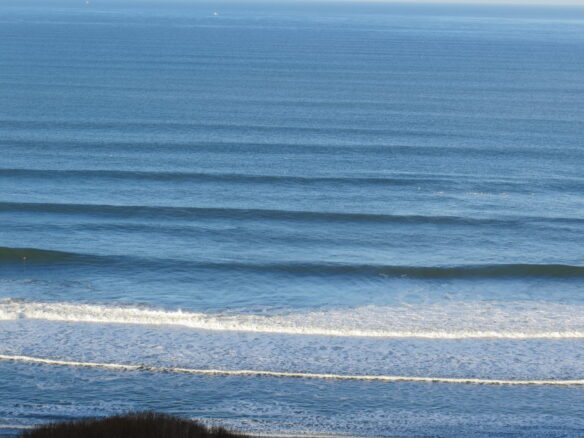
As the wind continues to blow during an offshore storm, the waves will gradually increase in height. It is the combination of the wind speed, the length of time the wind blows, as well as the distance over which it blows (which is called the fetch), that ultimately determine how large the waves can get. Through a combination of observations, measurements, and an understanding of how energy from the wind is transmitted to the sea surface, oceanographers that study waves have developed relationships between the wind conditions and the size of waves that can be formed. What has been discovered over years of studying waves is that in deep, offshore water the speed at which the waves travel is dependent on their wavelengths or their periods. The wavelength (L), if we are measuring in feet, is equal to 5.12 times the period (T) squared, or L = 5.12 T2. Thus a wave with a period of five seconds has a wavelength of 5.12 X 52 or about 128 feet. The velocity (V) or speed of the waves is also easy to calculate with V = 5.12T, so the same five second wave has a velocity of 5.12 X 5 or 25.6 feet per second, which is 17.5 miles per hour, faster than most of us can run. A 10 second wave, or a wave with a period of 10 seconds between each crest, is moving twice as fast or about 35 miles per hour at sea. The longest swell ever recorded had a period of 22.5 seconds and came out of the South Atlantic and measured along the Dover coast of England. Using the formulas above, these waves would have had a wavelength of half a mile and a velocity of 78 miles an hour!
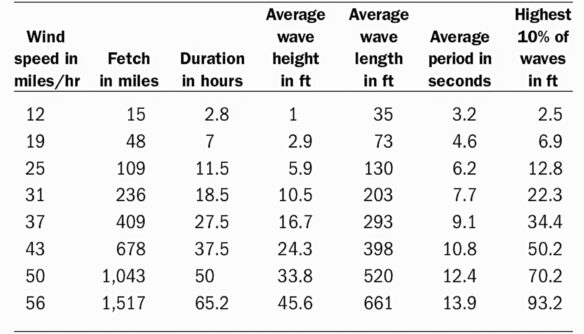
Because the speed of a wave is directly related to its length or period, the longer waves will move out away from the shorter ones during a storm simply because they are traveling faster. These longer waves may often be traveling faster than the storm front that created them and can arrive at the beach and create great surfing conditions some time before the wind and rain actually reach the coast.
While we say that the waves move or travel at a certain velocity, it is actually the form of the wave and not the water itself that advances across the ocean surface. If we watched some object floating in the water, or if you are out in the waves yourself, you will notice that as each wave passes by, you or the object you are watching will rise and sink and follow a circular orbit or path, returning to nearly the same place you started. The individual particles of water are following the same orbits and it is only the waveform that is advancing across the ocean surface. We can observe the same process by tying a rope to a post and moving the rope up and down to make waves in the rope. The rope itself doesn’t advance towards the post; it is just the waveform we have created that is moving. The wave that people create by raising their arms inside a sports stadium is exactly the same process. The people aren’t moving around the stadium, only the form of the wave is.
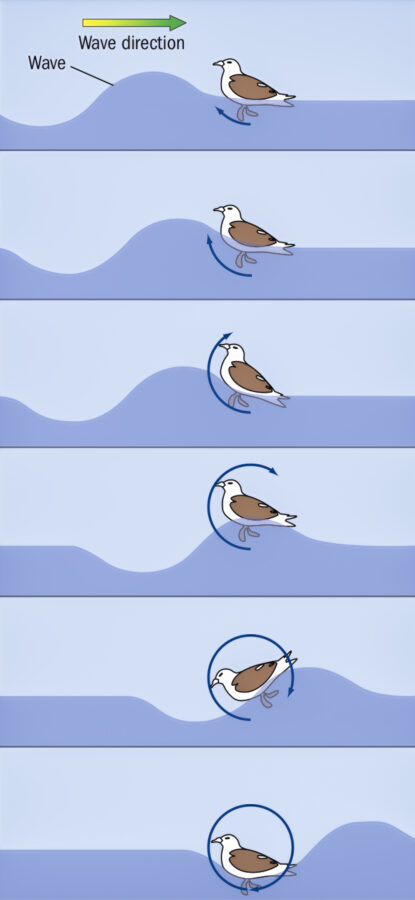
Waves formed during storms far out to sea in the Pacific, Atlantic or Indian Ocean will ultimately approach a shoreline where they will leave deep water behind, cross the shallow continental shelf, and begin to approach the beach. Depending upon the wavelength of the particular waves, they will begin to impinge on or feel the bottom somewhere on the shelf. Along the coast of California the shelf ranges in width from less than a mile off of Big Sur to over 25 miles off San Francisco. Along the US Atlantic or Gulf coast, the continental shelf is much wider, as little as 35 miles to well over a hundred miles.
As a wave passes through the ocean, it not only displaces tor oscillates the water at the sea surface but also moves the water at depth. This is why when you are diving under the waves or Scuba diving in shallow water, you will feel the water moving back and forth at depth. The depth at which water is set in motion by the wave passing overhead is equal to one-half of the wavelength. So to keep it simple, if the waves passing by at the surface have a wavelength of 200 feet, the circular motion involved with the passage of each waveform will extend to a depth of half of this or about 100 feet.
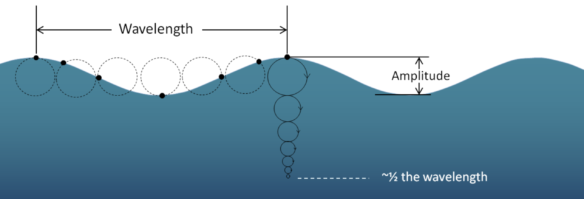
As soon as this deeper portion of wave begins to feel the bottom, it will begin to slow down due to friction with the seafloor, while the portion that we see at the sea surface continues to travel at its original speed. Several wave properties now begin to change because of this bottom friction. Due to the drag on the bottom as the wave continues towards shallower water, the overall wave velocity will begin to slow and the wavelength will begin to shorten as well. Because the circular or orbital motion of the water particles beneath the wave is being compressed or confined into shallower and shallower depths, the crest of the wave at the sea surface will begin to increase in height. While the waveform in the open ocean is a long series of broad swells and troughs, in shallow water this will change to a series of steeper and more distinct wave crests separated by flatter troughs. Eventually, the portion of the wave above the sea surface will become steep and unstable. When the ratio of the wave height to the water depth is about 3:4, the wave will finally break. Thus a 3-foot-high wave will break in about 4 feet of water, and a 6-foot-high wave will break in about 8 feet of water.
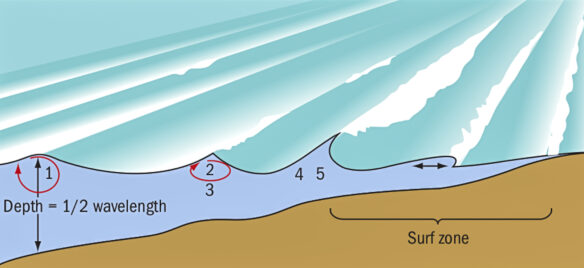
Waves can transmit enormous amounts of energy, and they are continuously being created while the wind is blowing, sort of an endless summer for wave formation. Thus, they are renewable, which is one reason why engineers and oceanographers have worked for years on schemes to utilize wave energy. To provide some sense of the power involved in waves, anyone who has seen storm waves batter a cliff, pier, or other oceanfront structure has witnessed the tremendous power they expend. A 10-foot wave can exert over 1,000 pounds of pressure per square foot, and wave pressures over 12,000 pounds or six tons per square foot have been recorded.
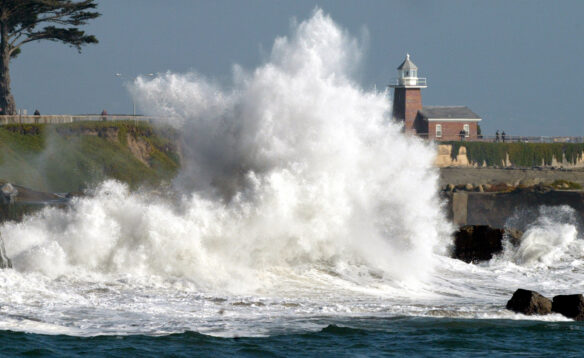
During a huge storm in 1914, wave run-up extinguished the Trinidad Head Light at an elevation of nearly 200 feet above sea level. The St. George Reef lighthouse near Crescent City experienced a massive storm in 1923 in which breaking waves swept over the foundation platform for the lighthouse, which was seventy feet above the water, tearing a large generator from its foundation. Restaurants along Cardiff’s restaurant row in northern San Diego County were severely damaged during the January–February 1983 storms when large waves hurled cobbles and boulders weighing up to 1,200 pounds into the buildings and onto the highway. These are just a few examples of the power of waves crashing on the coastline and is one major reason why anything built directly on the shoreline, whether on the cliff, dune, or beach, while providing great satisfaction for 6 to 9 months each year, is in a very vulnerable location every winter.
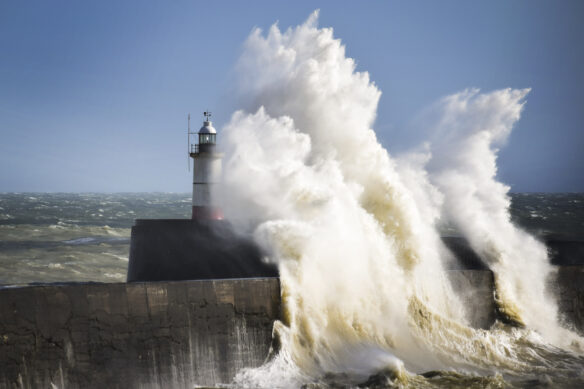
Sitting on a bluff or a beach somewhere watching the waves roll in, or being in the water itself, you will notice, most days, that the waves come in sets, or intervals, when the waves will increase in height and then get smaller again. If you are surfing, you may notice three or four large waves, when everyone attempts to take off, and then you might sit and wait for several minutes or longer for another set. You are observing the everyday process of wave interference. Along any stretch of coastline, there will usually be more than one group or train of waves arriving on any particular day, and they are probably coming from different directions, although that might be hard to tell while standing on the beach. Large waves may be arriving from some distant storm, while smaller waves are approaching from somewhere in the tropics, and then there may be a local wind swell from just offshore. As these different waves approach the shoreline, they will begin to interact or interfere with one another. This will produce a series of larger and smaller waves in some pattern that will be different every day depending upon the characteristics of the individual storm or local wind waves that are arriving. When the crests of two different wave groups are in phase and arrive at the same time at the shoreline, they will reinforce one another, or constructively interfere, producing higher than normal wave crests. If the waves are of nearly the same period, perhaps one group of waves being 10 seconds between crests and another being 11 seconds, then there may be a set of four or five waves that will form that are much higher than the others, providing good surfing conditions. The waves will then gradually move out of phase and the crest of one group of waves will arrive at the same time as the trough of the other group, and the waves will tend to cancel each other out as we observe destructive interference, with the resulting waves being much smaller or lower for a few minutes. Because the groups of waves that arrive on any beach will be different every day, there is no pattern or reason why the seventh wave or the ninth wave, or any other should be larger. It is a totally random process depending only on where storm waves are coming from, and the characteristics, such as period and height, of the different waves that arrive at any particular period of time.
Most waves arriving at the shoreline approach the coast at some angle rather than coming from directly offshore. Waves reaching the coast in the winter months along much of the California coast, for example, typically originate from storms in the North Pacific and, therefore, approach from the northwest. A south swell, or waves arriving from the Southern Hemisphere, is common during the summer months in southern and central California and may provide excellent surfing conditions at a time when waves are normally considerably smaller. Many of these waves are generated from storms off Antarctica, New Zealand, or Australia and have traveled thousands of miles from their origin.
Waves typically arrive at the shoreline in sets, periods of large waves followed by periods of smaller wave.
Think about a wave approaching the beach at an angle to the shoreline. As the water gets shallower near the coast, the portion of the wave closest to the beach “feels” the sea floor first and begins to slow down, while the seaward portion of the wave crest in deeper water continues to travel at its original speed. This results in the bending or refraction of the wave fronts as they approach the shoreline. As refraction continues, the wave fronts tend to become roughly parallel to the underwater contours. On an irregular, rocky coastline, refraction causes wave energy to bend around points or headlands, or shallow areas on the seafloor, thereby concentrating wave energy and increasing wave heights. The same bending or refraction process leads to wave fronts diverging in bays or over deeper areas such as submarine canyons, which leads to lower waves or energy dissipation. A mound on the shallow seafloor can then act like a lens to focus the wave energy and cause the waves to converge or increase in height passing over the mound. The refraction and, therefore, gradual breaking of the waves around a point or headland produces some of the best surfing spots in California, Malibu, Rincon, and Steamer Lane, to name a few. Surfing without wave refraction will produce very short rides.
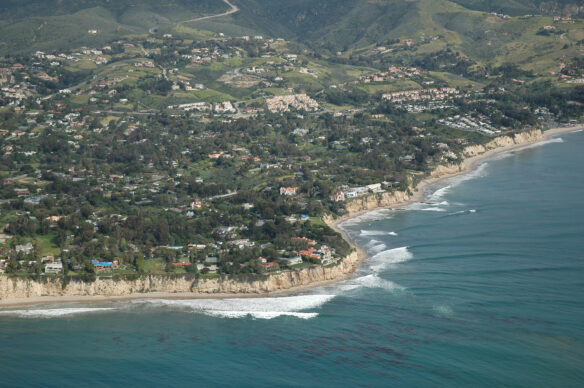
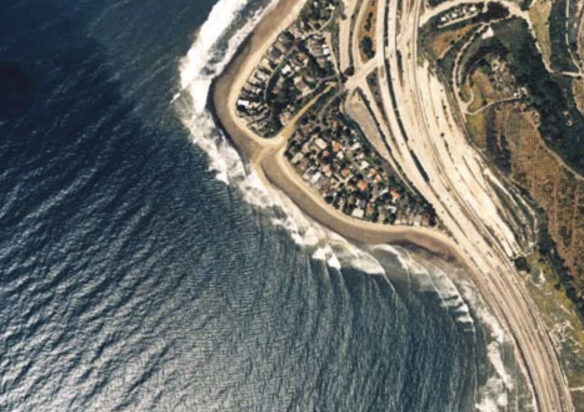
Winter and Summer Beaches
Anyone who has visited the same beach at different seasons of the year has probably noticed that the beach looks very different from winter to summer. Winter usually brings larger storm waves which tend to stir up the sand on the beach due to increased turbulence and carry it offshore where it is typically deposited as a set of bars and troughs. The beach will be narrower, lower in elevation and the beach will slope more gently. In some cases the beach may disappear altogether and reveal bedrock or coarser gravel or cobbles. With the late spring return to more gentle, less energetic summer waves, the sand that was carried offshore will be transported back onto the beach face, widening the beach and raising the elevation of the berm, or the high wide part of the beach. These conditions will usually dominate through the summer and into the late fall until the winter waves again return.
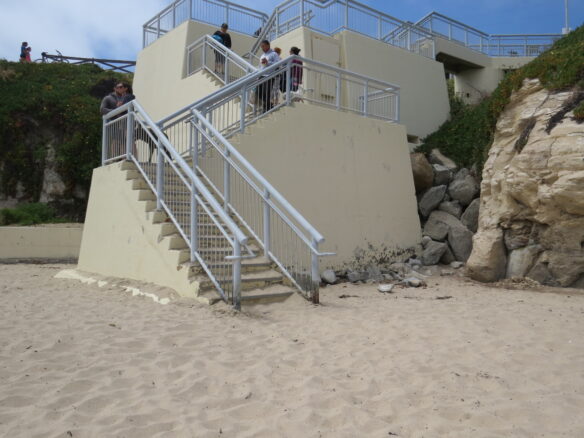
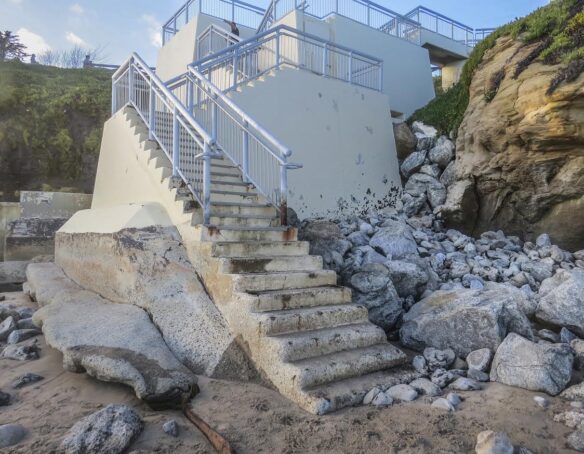
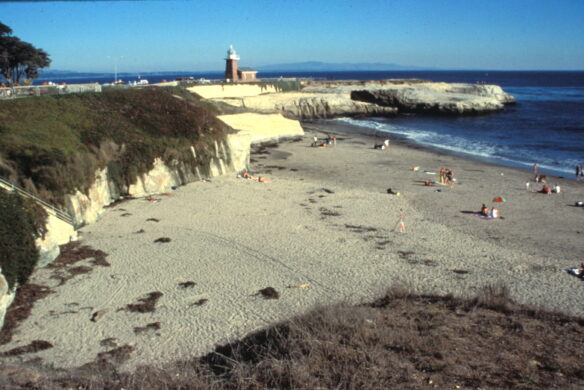
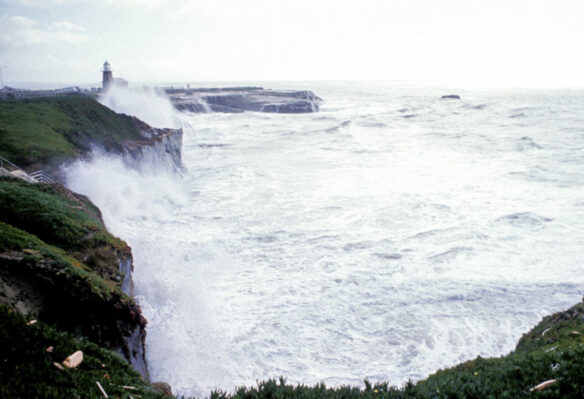
Those wide summer beaches produced by the less energetic summer waves provide several very important services, both a priceless recreational area but also a buffer or shock absorber protecting the cliffs, bluffs or dunes from wave attack. As mentioned earlier, winter waves can carry large amounts of energy and exert huge erosional forces on exposed cliffs and bluffs. Where beaches are wide, waves reach the base of the cliff or bluff much less often so erosion rates are much lower than for areas with narrow or only seasonal beaches. We need to protect and preserve our existing beaches and their sand supplies.