Green And Golden Seaweed Tides On The Rise, By Victor Smetacek & Adriana Zingone
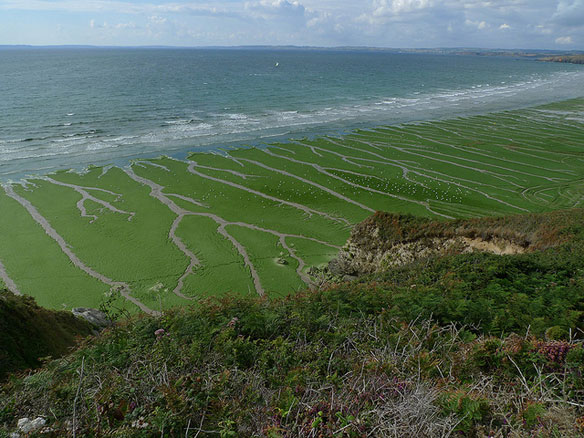
Green, brown and red seaweeds lying on the beach are part and parcel of life in many coastal regions. The amount of beached seaweed biomass started to increase along the shores of industrialized countries in the 1970s, and by the 1990s had become a nuisance along many beaches when mass-stranding events of macroalgae became known as green tides.
A Beach Project Built on Sand; By Robert S. Young, PhD
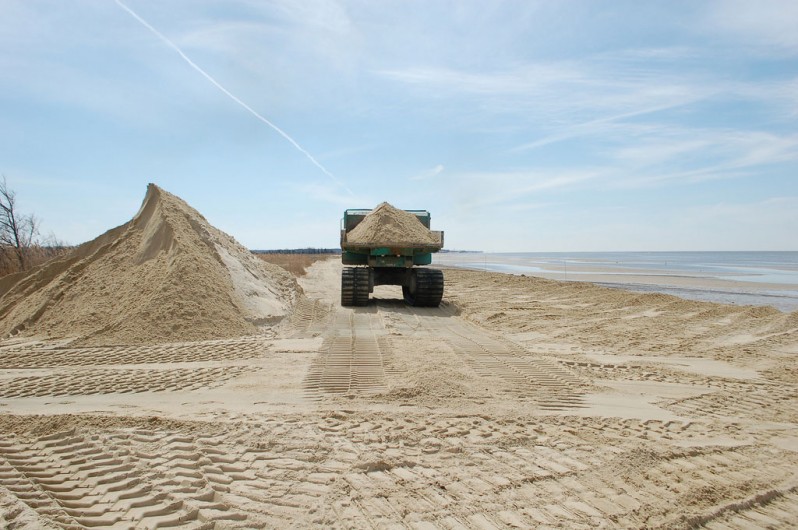
Earlier this month, Gov. Andrew M. Cuomo announced a $207 million plan to dredge millions of tons of sand off the south shore of Long Island and spread it along the beaches and dunes. It is a colossal waste of money and another consequence of the nation’s failure to develop a coherent plan to address the risks from storms faced by states along the eastern seaboard and gulf coast.
A New Frontier for Fracking: Drilling Near the Arctic Circle
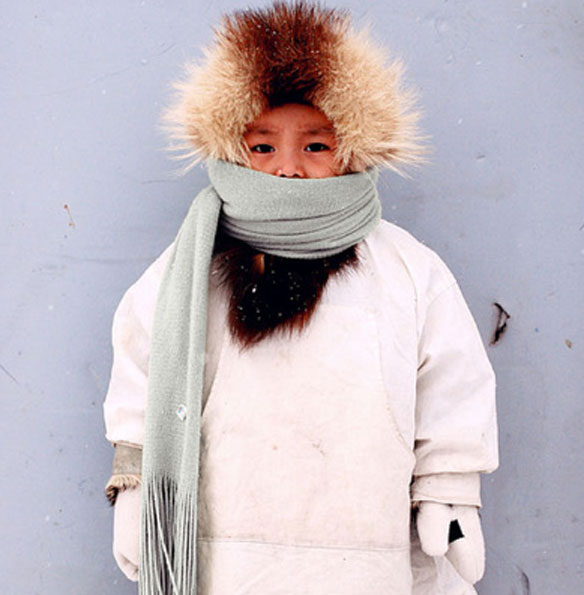
Hydraulic fracturing is about to move into the Canadian Arctic, with companies exploring the region’s rich shale oil deposits. But many indigenous people and conservationists have serious concerns about the impact of fracking in more fragile northern environments.
“El Expolio De La Arena”
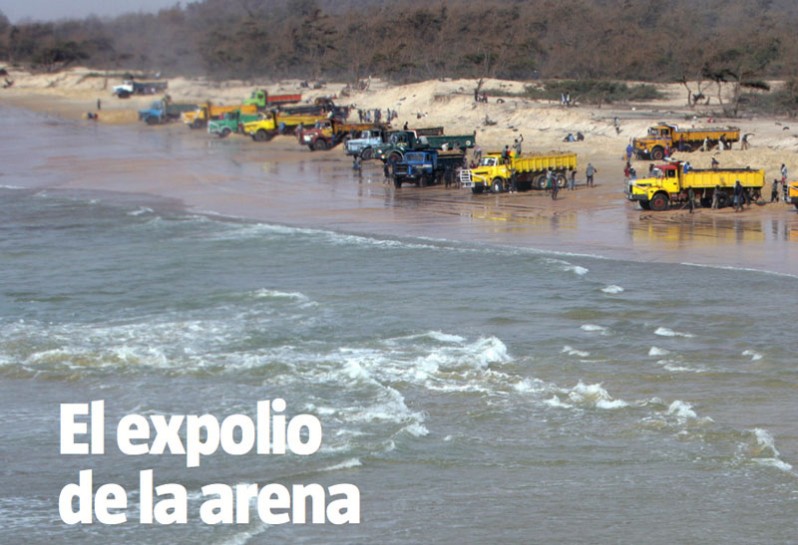
In this original version, investigative journalist Cristina Sáez, writing for leading spanish newspaper La Vanguardia, exposes how extensive, detrimental, silenced yet utterly pervasive “The pillaging of beach sand” has become.
City of Santa Barbara Sea-Level Rise Vulnerability Assessment: A summary Report
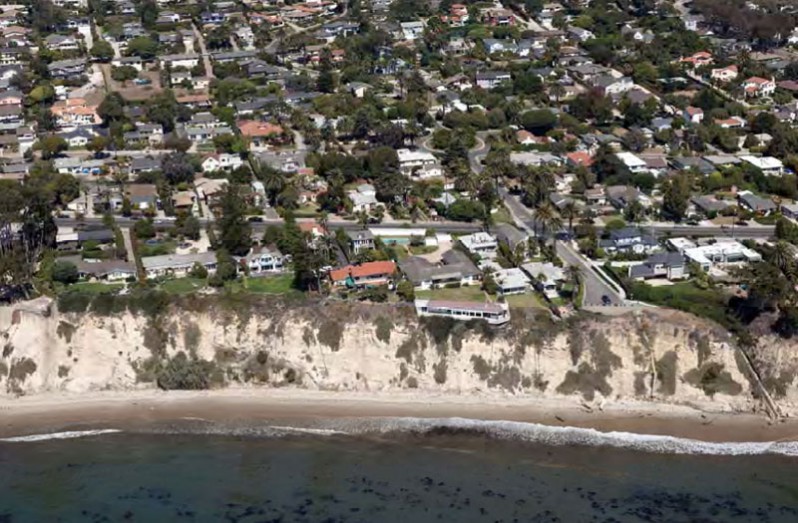
Gobal sea level is rising. As a result, many coastal communities will face tough choices for adapting to the future conditions and/or dealing with the consequences. The city of Santa Barbara faces a dilemma: Protect oceanfront development and infrastructure or remove barriers and let beaches migrate inland.
Why Wave Power Has Lagged Far Behind as Energy Source
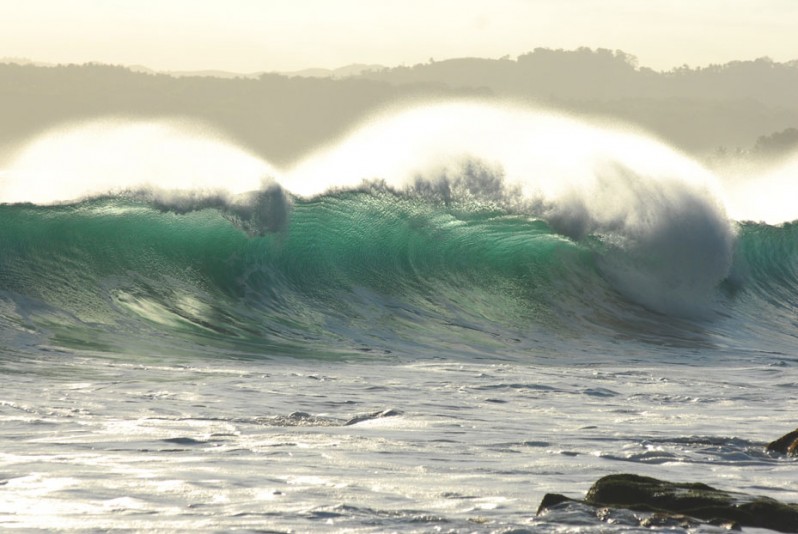
Researchers have long contended that power from ocean waves could make a major contribution as a renewable energy source. But a host of challenges have stymied efforts to generate electricity from the sea.
Call Climate Change What It Is: Violence
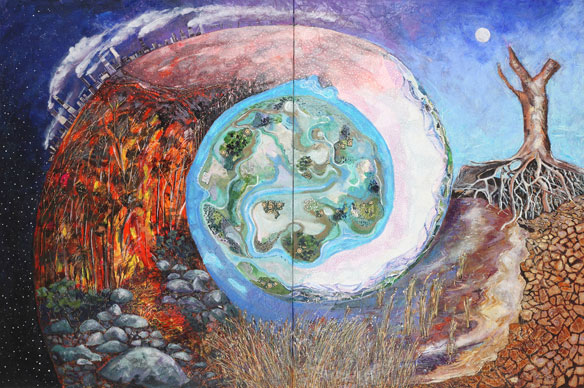
Climate change is global-scale violence, against places and species as well as against human beings. Once we call it by name, we can start having a real conversation about our priorities and values.
Coastal Barrier Resources System: Testimony of Robert S. Young, PhD
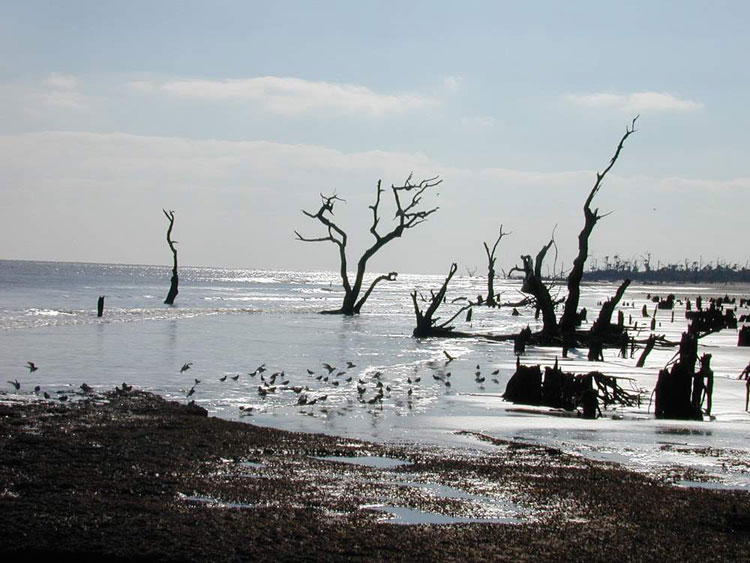
The Us Congress will hold a hearing tomorrow, April 8th, on 9 separate bills that would remove properties and lands from the Coastal Barrier Resources System, and Robert S. Young, PhD, PG, will be presenting his Testimony on the issue.
New UN Report Is Cautious On Making Climate Predictions
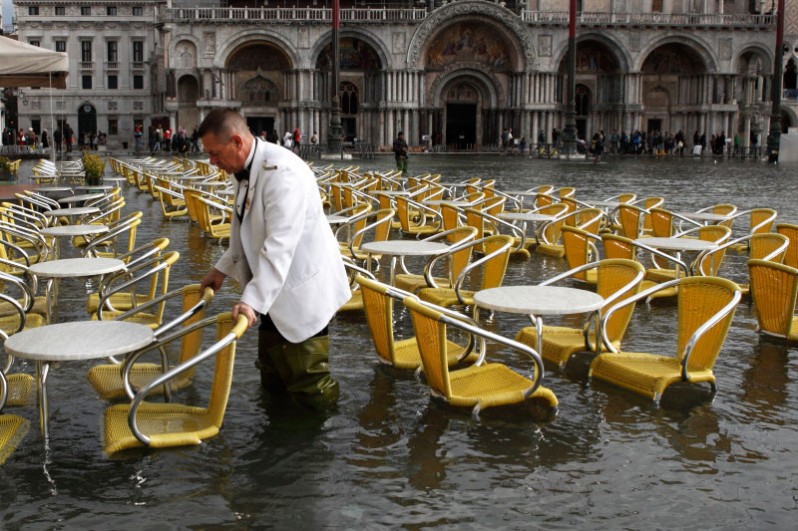
The draft of the latest report from the IPCC warns that the world faces serious risks from warming and that the poor are especially vulnerable. But it avoids the kinds of specific forecasts that have sparked controversy in the past.