Sand, Sea and as Much Serenity as You Could Ever Want – the New York Times
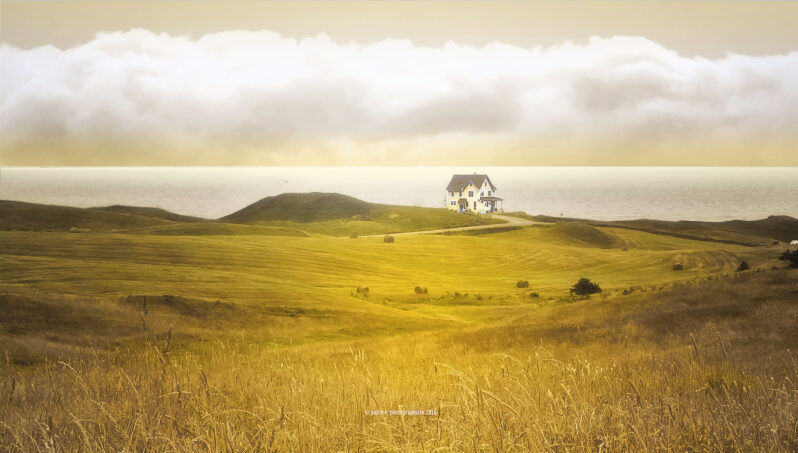
The bluffs, dunes and lagoons of the Magdalens, a colorful yet tranquil island chain north of Prince Edward Island, are far from everywhere. That’s the point…
10 Unique Beaches From Around the World – Atlas Obscura
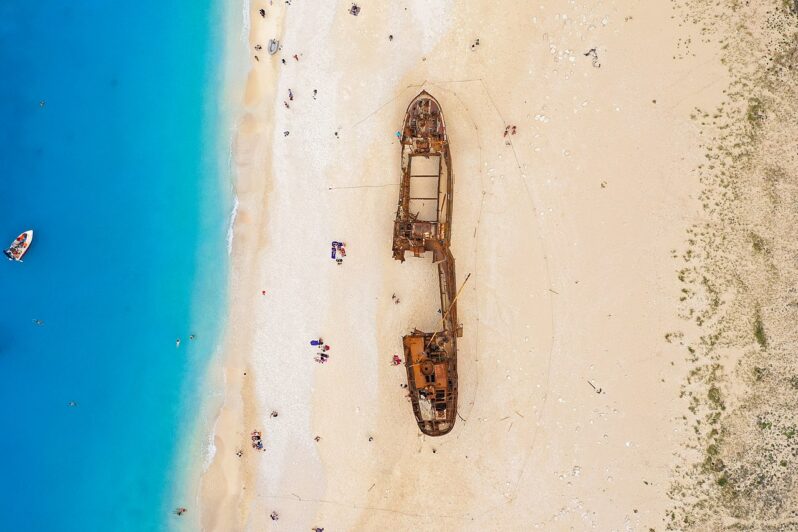
An otherworldly landscape of twisted trees in Georgia. A marshland of bright red grass on China’s Red Beach. A Wisconsin beach so beautiful, that taking rocks from it is illegal. An island of pink shores and Komodo dragons in Indonesia. If you’re looking for a unique beach, these ten places each offer something unexpected…
Finding a Way Back to ‘Perfect Beach’ – the New York Times
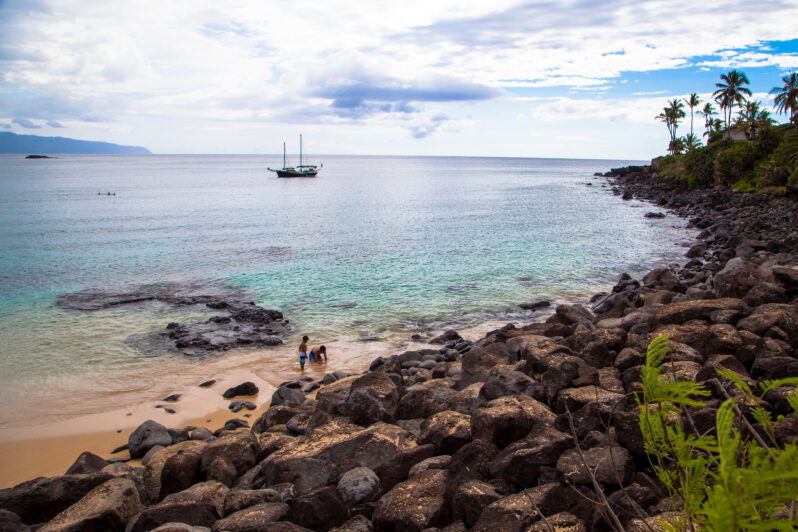
On the northern coast of Puerto Rico — about an hour’s drive west of San Juan, off a wisp of a road threaded through dense green foliage — there exists a long, empty beach that has haunted my dreams for years. On Google Maps, it appears as Punta Caracoles Beach, but I have always thought of it as Perfect Beach…
The Not-So-Natural Beach – Ryan Anderson
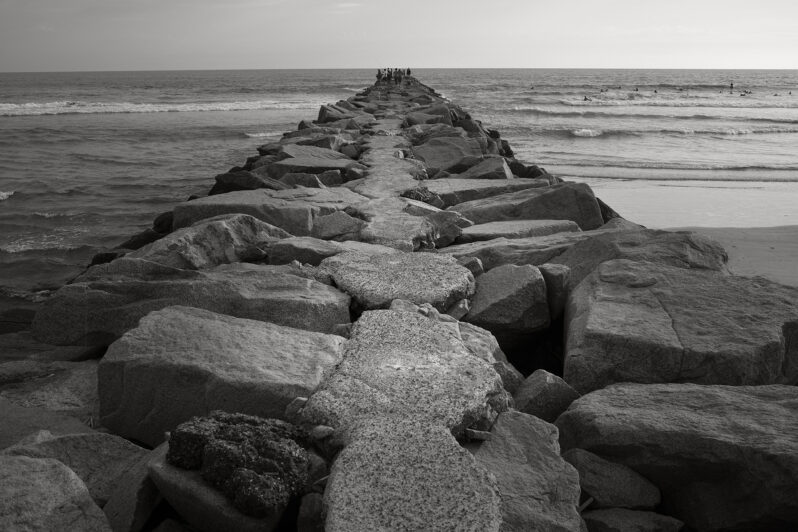
Growing up, I always imagined the beach to be a natural place. I think it’s safe to say that this sentiment may be pretty common among many beachgoers. It’s easy to think of the beach as being somewhat “natural,” or at least close to that thing some people call “nature.” This is a short piece, so I won’t go down the what is nature!? rabbit hole for now. By natural I mean something along the lines of “not caused or created by human intervention.” So here’s the thing: many beaches are actually far less “natural” than many people assume or know…
Two Beaches: Haukland and Uttakleiv Leknes, Lofoten, Norway
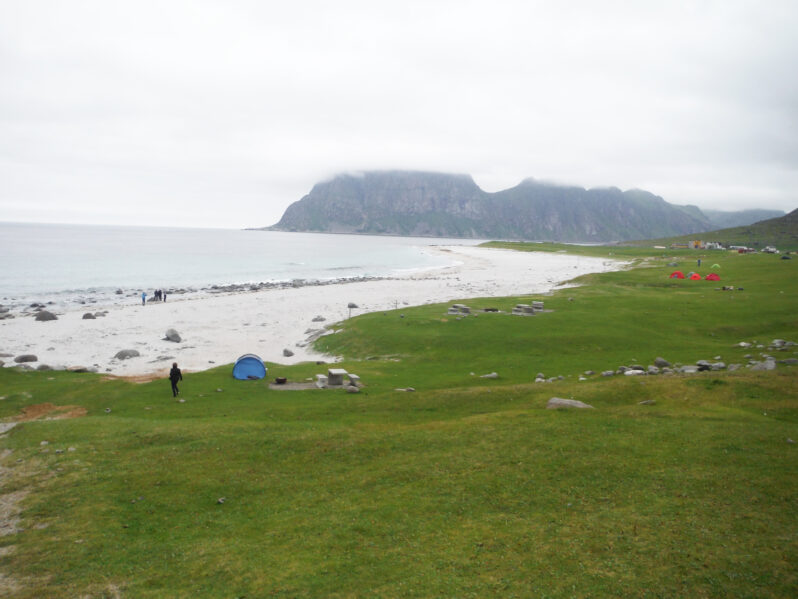
The Lofoten Islands make up an archipelago in the Norwegian Sea off the northwestern coast of Norway at a latitude of 68.1° N.
Beaver Island Michigan, 2016 – 2020: Beach Perspectives; By William J. Neal
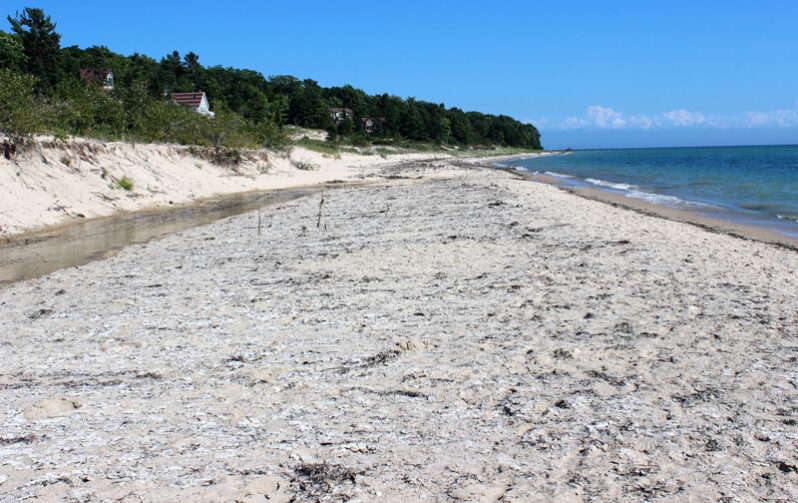
Most readers probably have heard of Mackinac Island, either for its strategic place in early American history, or as today’s high-end resort. In contrast, lesser known Beaver Island has a more colorful history.
Koekohe Beach and the Moeraki Boulders, South Island, New Zealand; By Norma Longo & Wayne Ranney

Not far north of the city of Dunedin is Koekohe Beach, a long, sandy beach on the east coast of the South Island of New Zealand, where a surprising and spectacular sight awaits. This beach is famous for its extraordinary boulders, the Moeraki Boulders.
Isla Arena: Where did this refuse come from? Marine anthropogenic litter on a remote island of the Colombian Caribbean sea
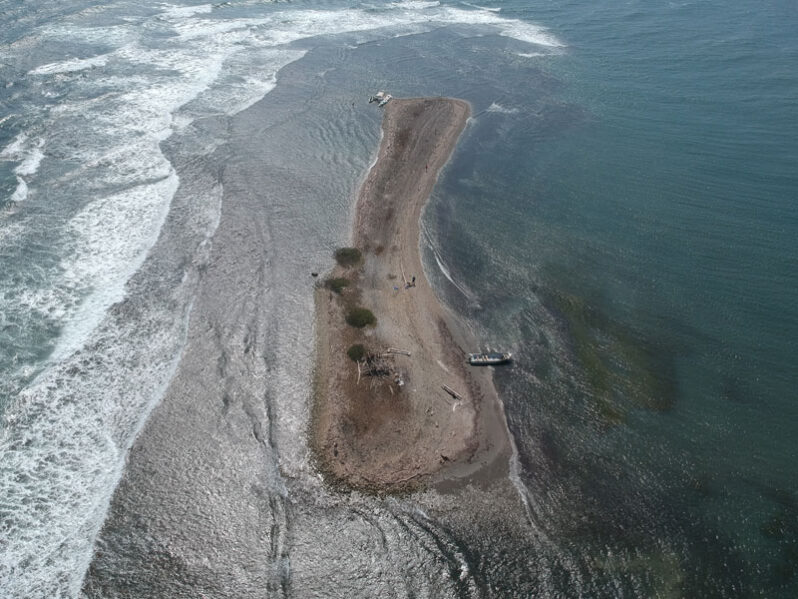
On the Colombian continental shelf, 12 km SW of the municipality of Galerazamba, Department of Bolívar, is the northern-most island of the Colombian Caribbean: Isla Arena. Despite being remote and uninhabited, this island is being affected by one of the most persistent problems in the marine environment: Marine Anthropogenic Litter
Coastal armoring and disappearing beaches; By Gary Griggs
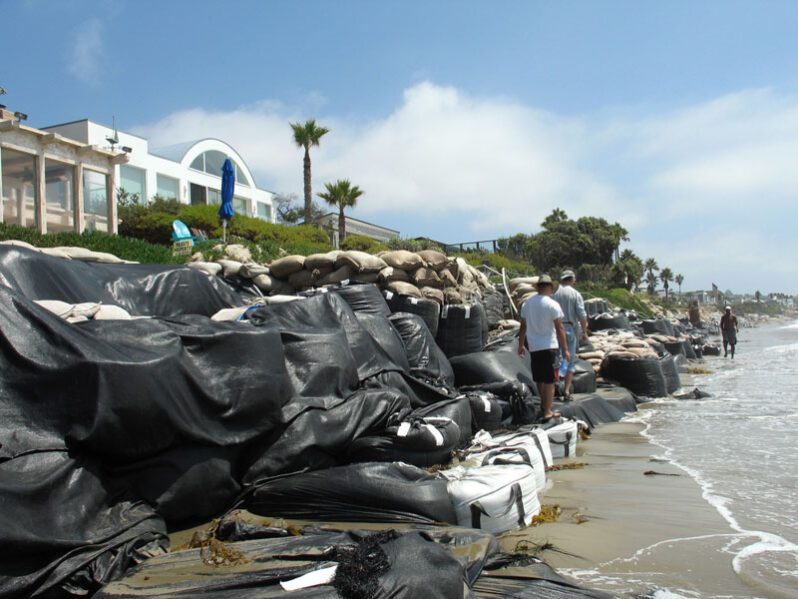
Coastal erosion or retreat is a natural ongoing process that has only become a problem because people have built structures or infrastructure in areas exposed to wave attack and erosion. Beaches, dunes, bluffs and cliffs are all temporary features that will continue to be shaped by wave, wind and tidal forces as well as a rising sea level.